SUMMARY
Composite spaced armor is an unconventional armor system capable of stopping armor-piercing (AP) projectiles at lower areal density than possible with traditional metallic and ceramic armor systems, which makes it especially attractive for weight-sensitive applications. Prior testing of this armor system at normal obliquity has shown that it has great potential to reduce weight in aircraft systems while providing improved ballistics protection. The anisotropic nature of this composite spaced armor further differentiates it from traditional metallic and ceramic systems because its directionally-dependent mechanical properties cause performance to vary with obliquity. Ballistics testing evaluated normal and oblique angle impacts to quantify performance at a range of shot lines. Results indicate that for a limited range of oblique shot lines, the tumble of the bullet is reduced, resulting in degraded performance of the armor. However, this can be mitigated through system-level design and careful integration or eliminated with technology solutions.
INTRODUCTION
In order to stop AP rounds, traditional armor systems require a hardened strike face, often made of ceramic material, which fractures the penetrator of the round. Including this hardened strike face results in a significant weight gain, making this type of armor impractical for weight-sensitive aviation platforms. A new composite spaced armor system, consisting entirely of ultra-high molecular weight polyethylene (UHMWPE), has been developed to defeat AP rounds at a much lower areal density than traditional systems.
Composite spaced armor is an unconventional armor system that uses two armor panels separated by an air gap. The first panel imparts an asymmetrical load onto the bullet, causing it to tumble. This rotation increases the presented area of the projectile and negates the advantage of the AP penetrator, allowing it to be stopped by the second panel. Spaced armor systems consist of three typical components—a striker, an air gap, and a catcher. In the case of composite spaced armor, the striker is an armor panel constructed out of UHMWPE using a proprietary manufacturing process. The trade name for this armor material is “turning block,” and it is manufactured by Hardwire LLC [1].
The bullet first passes through this striker panel, and the turning block imparts an asymmetrical load onto the round, causing it to tumble (Figure 1). This round then passes through an air gap, nominally ranging from 4 to 10 inches. A larger air gap can defeat faster rounds but is limited by the space allocated to the armor system. The tumble of the round increases the presented area of the projectile and turns the hardened penetrator of the AP round away from the path of the shot line. This rotation allows the catcher, also made of UHMWPE, to attenuate the energy and stop the round.

Figure 1: Turning Block Spaced Armor Integrated With Aircraft Skin and Floor Sections (Source: Robeson [2]).
PROBLEM/APPROACH
Traditional AP armor systems are metallic and/or ceramic; they exhibit a positive relationship between obliquity and performance. The more oblique the shot line, the more material is in the path of the bullet, and more energy can be attenuated. Such armor systems are isotropic and homogeneous—the mechanical properties are consistent throughout the material and behave the same in every direction. Composite armor systems, however, are anisotropic and nonhomogeneous—they exhibit different mechanical properties in different directions, and the properties change throughout the thickness of the material. This creates the potential that the armor system will behave differently at oblique shot lines compared to traditional metallic and ceramic armor systems, and there will not be a purely positive relationship between obliquity and performance.
In previous, unpublished testing of the subject composite spaced armor system, conducted internally by the Army, such a phenomenon was observed. At certain obliquities, the projectile tumbled consistently nose-up, while at other obliquities, the projectile tumbled consistently nose-down. The authors theorized that there is an obliquity that acts as an inflection point in which the striker imparts no tumble to the round and the armor system experiences degraded performance.
To test this hypothesis, ballistics testing was completed and analyzed at normal and oblique shot lines. Testing was conducted at an indoor range at the U.S. Army Combat Capabilities Development Command (CCDC) Aviation & Missile Center (AvMC) Ballistic Test Range for Aircraft Component Survivability at Fort Eustis, VA. A test rig was constructed (Figure 2, left) to hold the armor system in place with repeatable boundary conditions and simulate realistic shot lines. This setup enabled control over the obliquity of the striker and catcher, as well as the distance of the air gap.
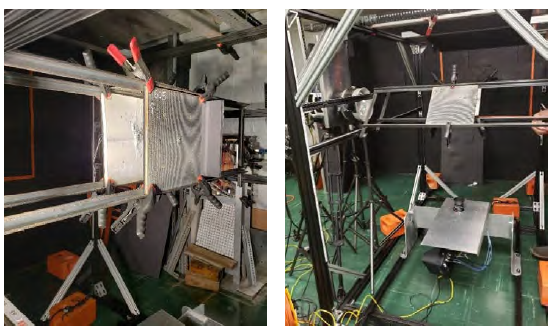
Figure 2: (Left) Test Rig at 0° Obliquity and (Right) Test Rig at 45° Obliquity With Cameras (Source: CCDC AvMC).
Two high-speed video cameras on the side and below the armor panels were used to record data (Figure 2, right). One camera captured video footage from the left side; this camera was used to measure the angle of the bullet’s tumble and its velocity as a function of distance. The second camera was placed on the bottom of the test rig and recorded video facing up toward the shot line; this camera was used to measure the yaw of the bullet. Both cameras were used in conjunction with a velocity screen, which measured muzzle velocity of the round directly after leaving the gun, to ensure accuracy of the high-speed video velocity measurements. The video consistently measured 1.1% slower than the velocity gates; this discrepancy was considered acceptable.
Two types of tests were conducted to better understand this armor system’s performance. The first test was a standard V50 determination, as specified by MIL-STD-662F [4]. A V50 describes the velocity at which an armor has a 50% chance of stopping a given projectile (threat); thus, a higher V50 is desirable for a given weight. Second, turning block was shot without a catcher at varying obliquities and with constant velocity (100-m standoff velocity ±100 ft/s). This test examined the round’s angle of tumble as a function of both air gap distance and armor obliquity.
The areal density of the armor, the threat being tested, and the velocity of the round will not be disclosed in this article due to their security classifications. However, in order to portray the effects that velocity had on test results, this classified velocity (which corresponds to the 100-m standoff velocity of the unstated threat) will henceforth be referred to as the “reference velocity.” Each subsequent velocity included here will be a delta (Δ), or numerical difference, from this unstated reference velocity.
RESULTS
V50 Testing at 0° and 45° Obliquities
First, V50’s values were determined at 0° and again at 45°, each test maintaining a 6-inch air gap between striker and catcher. The results can be seen in Figures 3–6. Figures 3 and 4 demonstrate the relationship between the angle of tumble and the distance traveled from the back face of the striker, while Figures 5 and 6 illustrate the relationship between bullet velocity and angle of tumble. Additionally, color coding is used to differentiate between shots that were a partial penetration (green) vs. complete penetration (red). A partial penetration is terminology used by the armor community to specify a successful stop, but one in which the armor accrued some damage.

Figure 3: V50 at 0°: Angle of Tumble vs. Distance Traveled (Source: CCDC AvMC).

Figure 4: V50 at 45°: Angle of Tumble vs. Distance Traveled (Source: CCDC AvMC).

Figure 5: V50 at 0°: Velocity vs. Angle of Tumble at 5 Inches (Source: CCDC AvMC).

Figure 6: V50 at 45°: Velocity vs. Angle of Tumble at 5 Inches (Source: CCDC AvMC).
A few preliminary conclusions can be drawn from these initial V50 tests. First, the performance of the spaced armor system was fairly consistent at normal obliquity. The relationship between angle of tumble and the distance traveled was mostly linear, leading to the conclusion that the turning block imparts a constant tumble rate on the round.
Bullet velocity, as well as angle of tumble, both affect whether the shot is a complete or partial penetration. As the velocity increases and/or the tumble decreases, it appears that the round becomes more difficult to stop. This conclusion follows the principles that govern spaced armor performance. A faster round has more energy, and a less-tumbled bullet has a lower presented area, resulting in greater impact energy per unit area. Both conditions decrease the effectiveness of the catcher. It is worth noting that there are multiple causes of reduced tumble angle, including faster velocity (less time to tumble) and smaller air gap (less distance to tumble).
Normal obliquity (0°) produced a V50 of Δ5.8 ft/s (regarding reference velocity), and 45° resulted in Δ147.3 ft/s. The V50 at 45° was higher than the V50 at normal obliquity; however, the predicted anomaly was observed in the course of testing. Ten shots were fired to calculate the V50 at 45°. One of them exhibited almost no tumble, causing it to pass straight through the armor system with little attenuation of energy. This particular shot can be clearly distinguished in Figure 4 as the line with 0° slope (the angle of tumble does not increase with distance traveled). This degraded performance condition is considered an inherent characteristic of the spaced armor system, so the anomalous shot was classified as a valid data point (not an outlier) and is included in the V50 calculation.
While 0° tumbled nose-down 100% of the time (12/12), 45° tumbled nose-up ~90% (9/10) of the time. After seeing 1/10 shots exhibit this decrease in angle of tumble, it was clear that some degraded performance in this spaced armor system was present. The angle of the armor system was then stepped back first in 5° and then 10° increments from 45° to 15° to determine if more pass-through events were possible.
Angle of Tumble vs. Distance Traveled at Varying Obliquities
In these tests, the gunpowder grain input was modified to shoot all of the projectiles at the reference velocity to hold this variable constant. However, due to the variable nature of ballistics, a delta of ±100 ft/s is present in this data. Additionally, the rounds were shot at turning block only; no catcher was used to observe the angle of tumble past the traditional air gap maximum distance of 10 inches.
Various obliquities were tested, and the results are shown in Figures 7–11. Additional 0° and 45° data were captured without catchers, and the results are included in this set. Figures 10 and 11 show the angle of tumble as negative, which corresponds to the bullet tumbling nose-down instead of nose-up.
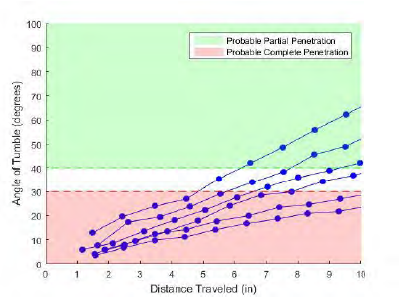
Figure 7: 0°: Angle of Tumble vs. Distance Traveled (Source: CCDC AvMC).

Figure 8: 30°: Angle of Tumble vs. Distance Traveled (Source: CCDC AvMC).
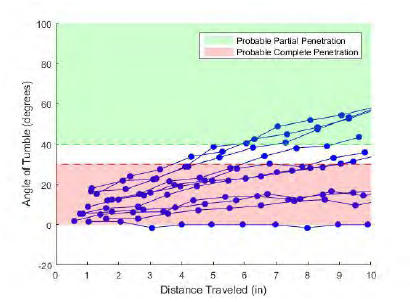
Figure 9: 35°: Angle of Tumble vs. Distance Traveled (Source: CCDC AvMC).
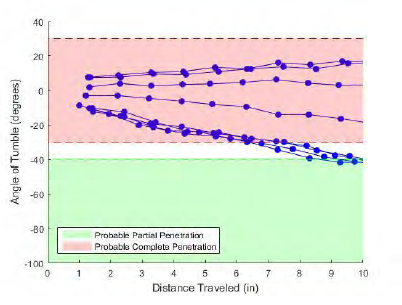
Figure 10: 40°: Angle of Tumble vs. Distance Traveled (Source: CCDC AvMC).

Figure 11: 45°: Angle of Tumble vs. Distance Traveled (Source: CCDC AvMC).
The coordinate system is relative to the orientation of the turning block; in this experiment, it was set so that every bullet tumbles nose-up at normal (0°) obliquity. Analysis of the previous V50 test results indicates that 100% of bullets fired were stopped when they exhibited at least ±40° of tumble. Therefore, shots that meet this criteria are assumed to result in a partial penetration (represented in green in Figures 7–11).
Alternatively, according to analysis of previous V50 tests, 100% of bullets fired passed through when they displayed less than ±30° of tumble. Therefore, it was assumed that shots meeting this criteria would result in a complete penetration (shown in red in Figures 7–11). The section of the plots with no color coding, between ±30° and ±40°, represents an area where there is no conclusive evidence to predict how the armor will perform based on current data.
After analyzing the test results, 40° appears to be the hypothesized inflection point. As shown in Figure 10, some shots exhibit a nose-up rotation, others exhibit little-to-no tumble, and the rest exhibit a nose-down rotation. Due to the variability and lack of rotation of the projectiles at this obliquity, it would be expected that the performance of the armor system as a whole would be reduced.
To further characterize the performance of the armor system at the 40° obliquity, V50 testing was conducted; the results are displayed in Figure 12. The air gap was initially set to 6 inches, but no partial penetrations occurred within four shots, even after significantly reducing the velocity to Δ765 ft/s below the reference. At this point, the air gap was increased to 10 inches; however, the armor system was still unable to stop the round. After five shots, this V50 test was concluded because it was clear that a worst-case obliquity was identified.
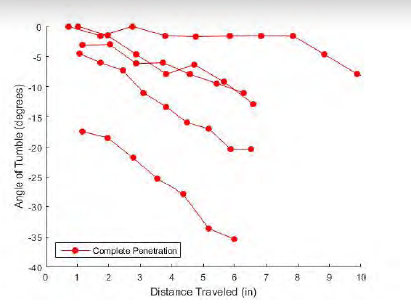
Figure 12: Attempted V50 at 40°: Angle of Tumble vs. Distance Traveled (Source: CCDC AvMC).
CONCLUSIONS
While more work and testing needs to be done to make statistically-stronger conclusions, preliminary trends can be drawn from this experimental data. At normal obliquity, this armor system performs, as intended, against the proposed threat at 100-m standoff velocity and is effective at stopping the projectile at much lower weights than traditional armor systems.
As hypothesized, there appears to be an inflection point, at or near 40°, where the tumble of the round is greatly reduced, resulting in degraded performance. Additionally, the obliquities near the inflection point, especially between 30° and 40°, also exhibit similar intermittent reduction in tumble and decreased performance.
At and around the 40° inflection point, there were certain shots that exhibited little-to-no tumble. These shots were especially noteworthy, as bullet rotation is the only mechanism by which this spaced armor system can defeat AP threats at a reasonable areal density. While this undesired phenomenon did not occur 100% of the time, its existence, even intermittently, threatens the performance of the armor at this specific, narrow range of obliquities.
To fully characterize this armor system, additional research and testing are needed to better understand when and why this reduced tumble occurs and the full impact of the lack of tumble. This increased understanding will enable the development of solutions to overcome this inherent vulnerability in the armor. It is worth noting that these solutions, while likely able to eliminate the inherent vulnerability, will probably increase the overall weight of the armor. However, this vulnerability can also be mitigated through system-level design and careful integration without any need to add weight to the system.
While the armor system suffers from a small range of vulnerable shot lines, it represents a new, effective, lightweight class of high-performance armor. Its multifunctional applications and low areal density make it attractive for aircraft, as well as ground vehicles and building walls. Further development work could enable such systems to revolutionize Army rotorcraft survivability.
REFERENCES
- Tunis, G. C., S. Kendall, and S. L. Kinnebrew. U.S. Patent 8,739,675 B2, 2014.
- Robeson, M. “Structural Multifunctionality for Weight Reduction.” Proceedings of the AHS 74th Annual Forum, Phoenix, AZ, May 2018.
- Berry, O., D. Misciagna, G. Hickman, M. Molitor, and B. Justusson. “Highly Durable Floor/Armor for Rotorcraft (HDFAR) Program: Phase 6 of the Rotorcraft Durability and Damage Tolerance (RDDT) Program.” Final Report for Agreement W911W6-11-2-0014, U.S. Army Research, Development and Engineering Command Technical Report (TR) 18-D-63, June 2019.
- U.S. Department of Defense. V50 Ballistic Test for Armor. MIL-STD-662F, revision F, 18 December 1997.