SUMMARY
This article addresses some of the challenges associated with explosive ordnance disposal (EOD) techniques when applied to insensitive munitions (IMs). The established disposal techniques for conventional munitions or unexploded ordnance (UXO) typically involve high-order, sympathetic detonation caused by bare donor charges or applying specialized EOD shaped charges. Depending on design, shaped charges can induce either high- or low-order detonation when used with conventional munitions. These techniques have shown limitations when applied to IM. The gap between the energy needed to achieve case penetration to enable access to explosive fill and the full initiation of the munition may be more difficult to achieve for insensitive high explosive (IHE)-filled UXO that have been exposed to violent stimuli, partially armed, armed, or partially functioned from failure to function as designed. The process of establishing new EOD methods for use against IM will require techniques used to achieve high- and low-order reactions. This may be achieved by modifying techniques historically used for conventional munition disposal if developers can overcome the limitations of these techniques when applied to IM. This article discusses challenges associated with developing new IM EOD disposal methods and presents examples of existing and emerging technologies that may offer possible solutions.
The established disposal techniques to conventional munitions or unexploded ordnance typically involve high-order, sympathetic detonation caused by bare donor charges or applying specialized EOD shaped charges.
BACKGROUND
In order to fulfill required missions, the U.S. Armed Services need to procure weapons with a high degree of effectiveness in sufficient volume for sustained combat operations while ensuring the greatest degree of safety as reasonably achievable to personnel and logistic assets. One way to support this goal is to design munitions that do not react violently to inadvertent stimuli. This approach presents an added benefit by complicating reuse by adversaries. As modern battlefields increasingly shift into populated urban centers, IM inventories represent a less-desirable target for terrorists and minimize the threat to surrounding communities. Insensitive munitions could potentially be more cost effective to transport if the goal of lower U.S. Department of Defense (DoD)/Department of Transportation hazard classification rankings can be achieved. In addition, the reduced probability of catastrophic accidents involving IM allows more munitions to be stored in a given area because of reduced, mandated quantity-distance separation requirements.
Developing appropriate procedures for disposal of explosive ordnance is mandated by Allied Explosive Ordnance Disposal Publication 10 and echoed in North Atlantic Treaty Organization (NATO) Standardization Agreement (STANAG) 2143 [1]. As a result of IM programs, many munitions that are increasingly less shock sensitive and have increased critical diameters are being fielded. This creates problems for EOD technicians while conducting a render-safe procedure and ordnance disposal procedures. Traditional EOD methods consist of a number of historically proven techniques—shock initiation by donor charge, directed energy attack (e.g., shaped charge), thermal initiation charge (thermite), and stand-off munitions disruptors. In addition, UXO disposal operations may require a nondetonating response when the situation will not allow high-order detonation disposal. As newer insensitive munitions are designed to prevent continuation of a violent reaction to external stimuli, achieving a complete or nearly complete low-order reaction presents its own unique set of challenges. Additionally, recent experience has highlighted the requirement to remove explosive ordnance from the operational theater to deny its reuse in improvised explosive devices. This has led to significant dedication of resources in theater that utilize expedient methods of disposal.
In 2017, the Joint Service EOD Program Office completed an Analysis of Alternatives on IM disposal. While there is a commitment to remaining informed regarding what is being developed in the IM community, no specific path forward to addressing EOD needs for IM disposal was chosen at that time.
DISPOSAL PROCEDURES
Disposal of IM is an emerging issue in need of attention throughout the defense industry, governments, and humanitarian demining organizations. In 2012, the DoD used the Small Business Innovation Research program to solicit proposals for technology that can reliably cause detonating IM and/or bulk IHEs without large amounts of donor explosives [2]. Numerous DoD agencies, national labs, and defense contractors have, or are currently investing in, IM disposal technology development programs; however, little has been published in open forums. The NATO EOD Working Group has demonstrated interest but has not yet issued NATO guidance for IM disposal procedures.
Sympathetic Detonation
Sympathetic detonation is a disposal method that uses the influence of a donor explosive placed nearby to initiate a detonation in the target munition. Large IM-filled munitions present difficulties for disposal by open detonation due to the large amount of donor explosives required to initiate the munition and the risk of incomplete consumption of the explosive fill. Traditional methods of sympathetic detonation for conventional munitions by direct application of bare donor, high-explosive charges have failed to reliably and repeatedly initiate and/or consume insensitive explosive fills during emergency destruction. Initial modifications of existing techniques required up to 400% more donor explosives and typically achieved only partial detonation. The increase in total explosive weight can exceed range limits previously set by conventional munition disposal allowances. Where munitions can be disposed by open detonation, incomplete destruction with large pieces of unreactive energetics typically results. This can create significant logistical hurdles, health, and safety concerns for explosive ordnance disposal personnel.
Countercharging IM-compliant, small-diameter munitions with large volumes of bulk conventional high explosives may lead to effective disposal. However, this can present a significant logistical burden and an unnecessary consumption of explosives. It may also increase the overall explosive hazard. These hazards are multiplied for disposal operation in high-threat environments. Experience from combat theater operations has demonstrated the requirement for economy of effort and the importance of limiting the risk to EOD operators.
Shaped Charge Attack
Manufactured shaped charge EOD tools capable of producing sufficient diameter jets have become an impractical proposition for dismounted EOD operations. A trend in recent years has been developing commercially-provided, user-filled shaped charge systems and, in some instances, offering a variety of liner materials and configurations. This approach has value in reducing the logistical burdens associated with prefilled, manufactured EOD charge systems. Reliability and repeatability of the explosive effect from the tool and target munition response will dictate acceptance from the EOD community. Shaped charge attacks also have potential use for low-cost, planned demilitarization of IM.
Commercial Shaped Charges
Commercial products include various-sized, shaped charges, some with specific application such as underwater demolitions. Both manufactured, flexible, linear shaped charges and hand-packed, high-explosive shaped charges have yielded predictable results when applied to IM regarding warhead case rupturing and segmenting rocket motors.
Thunder Range (organization 6647) of Sandia National Laboratories, NM, recently demonstrated the Cylindrical Dynamic Access Tool (CDAT) to be an effective means of disposal of certain IHE munitions using 1 lb of hand-packed explosives (see Figure 1). This technique can be improvised by the user, or the plastic body may be purchased through a commercial vendor (Team Technologies, Albuquerque, NM). The CDAT’s production plastic body is a user-configurable, multiuse charge with advanced capabilities, including a shaped charge, an explosively formed penetrator, and a contact charge. Energetic materials required to fill it are PETN-based sheet explosive and plasticized, moldable explosives like C-4 or Symtex.
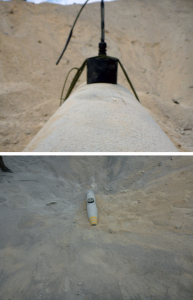
Figure 1: Placement of Cylindrical Dynamic Access Tool Prior to Initiation (Source: Sandia National Laboratories).
The Alford Technologies’ Krakatoa is a 100- x 100-mm charge container body with two load position options to accommodate either 500 or 1000 g of plastic explosive (see Figure 2). The main body of the larger Vesuvius charge is approximately 160 mm long, 160 mm in diameter, and capable of holding ~4 kg of plastic explosives. The size and performance characteristics of the Vesuvius make it a suitable surrogate for testing armor against improvised, explosively-formed penetrators. No open-source test data are available assessing these commercially-available tools’ performance against IM targets [3].
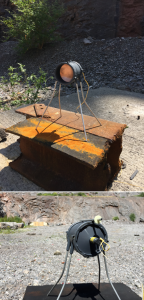
Figure 2: Alford Technologies’ Krakatoa disruptor (Source: Alford Technologies).
Northrop Grumman (formerly, ATK Ordnance and Ground Systems) produces the SM-EOD family of explosive charges consisting of RDX, wax, and graphite, initiated with a No. 8 or equivalent blasting cap. The SM-EODs are manufactured in accordance with MIL and NATO standards and available in six calibers.
Shaped Charge Jet Attack
The response of a munition attack by a shaped charge jet depends on many factors, including characteristics of the fill, confinement, case thickness, and jet tip velocity and diameter. If a jet is sufficiently fast, its penetration velocity will exceed the explosive’s detonation velocity and the technique may achieve a steady-state detonation. Lower-velocity jet results in slower penetration, where the detonation wave precedes the penetration process to consume the explosive. Achieving sufficient penetration to ensure complete consumption of insensitive fills without initiating a high-order detonation requires complex geometry specifically tailored to the target munition.
It is possible to determine the approximate charge diameter necessary to reliably initiate insensitive explosives through a shaped charge jet attack given the jet tip velocity, critical diameter of the explosive fill, and case composition and thickness. Held calculated a minimal threshold value for initiating explosives to be a product of the jet tip’s velocity squared multiplied by the jet tip’s diameter [4].
Patel and Voisin have shown that Held’s criteria values correlate to critical diameters [5, 6]:
Pope and Baker calculated the diameter of the jet tip to be approximately 0.04133 x the diameter of the shaped charge [7]:
By using the known jet tip velocity and compensating for decreased velocity due to case penetration, a rough estimate can be determined for the size of the shaped charge needed to initiate a given munition. For example, Pope and Baker calculated a minimal shaped charge diameter for an IMX-101 charge at critical diameter [7]. Their results are summarized in Table 1.
Table 1: Held’s Criterion Used to Predict Shaped Charge Diameters [8]
Majerus reported developing advanced shaped charges with roughly doubled jet diameters than those produced by conventional shaped charge designs of the same size [9]. Currently, the shaped charge liners produce large-diameter sections at lower jet velocities. However, modifying the shaped charge design to achieve the necessary jet tip velocity at increased jet tip diameter is possible. An advanced shaped charge design that produces roughly doubled jet diameters could potentially halve the required shaped charge disruptor diameters.
Shock Loading
The response of energetic materials to low-amplitude/long-duration impact and shock loading may have potential application for disposal of IM. It is possible that an initial shock-loading event (e.g., impact flyer plate) could sensitize an insensitive fill to subsequent impacts, which may then lead to a more violent reaction than would have occurred in response to a single flyer plate impact. Experiments by Haskins et al. demonstrated that detonation via an unknown detonation transition or deflagration-to-detonation transition process may be produced through intentionally applying multiple fragment impacts [10]. A related phenomena using colliding shock waves generated by two simultaneously-initiated bare donor charges is under investigation as a possible technique for IM disposal.
Explosively-Generated Plasma (EGP)
EGP is created by focusing explosive ejecta through a conical waveguide (e.g., Imhoff cones) that compresses the gasses to the extent where plasma is produced. Plasma can travel at velocities as high as 21,000 km/s, while producing temperatures as high as 20,000 K [8]. The Naval Surface Warfare Center Indian Head Explosive Ordnance Disposal Technology Division demonstrated that plasma can penetrate ordnance casing without fragmentation or deformation through the plasma ablation process. When plasma interacts with the munition case, the energy is transformed into heat, leading to melting and evaporating the case material at the interaction point. After penetration, the EGP causes a high-temperature chemical decomposition, resulting in deflagration of conventional energetic fills.
MUNITION MARKING
Positive identification of all UXO is required prior to performing a render-safe procedure or disposal operations. Recently ratified changes to Allied Ordnance Publication (AOP) 2, the NATO standard that governs marking munitions, include the requirement for marking the explosive name or official designation by stenciled or permanent marking every 90 degrees around the body [11]. This is mandatory for all newly-manufactured munitions from 20-mm and larger-caliber munitions, including aircraft bombs. The U.S. Joint Ordnance Technical Publication (JOTP) 70 amends this NATO requirement to only identify munitions with less-sensitive explosive fills [12].
Additionally, JOTP-70 provides alternate marking options if the markings negatively impact IM mitigation measures such as the thermal protective coating. In these instances, the marking may be applied to a less-vulnerable part of the munition, typically engraved in the baseplate. These updates will assist EOD technicians in distinguishing IM from their more sensitive counterparts and apply appropriate tools and techniques for disposal operations. Preliminary research efforts have studied using radio frequency ID tags for artillery rounds so that EOD teams can unambiguously identify UXO.
HEALTH CONSIDERATIONS
Part of the development of any new weapon system is considering toxicology of new formulations and its life-cycle impact from raw materials, manufacture, use, and disposal. Initial estimates for potential health impacts to EOD personnel were derived from exposure rates established through studies of conventional munitions disposal. Field testing munitions for energetics residue deposition demonstrated that a significant portion of some energetic compounds remained after detonation. Also concerning is that conventional disposal techniques applied to next generation IM often fail, resulting in releasing wholly-unreacted or partially-reacted fills and potentially increasing exposure and resulting health complications.
A significant amount of work has been dedicated to measuring the amount of explosives remaining on the ground following the high-order detonation of conventional explosives. In May 2012, Strategic Environmental Research and Development Program (SERDP) investigated the life-cycle environmental impacts of the first generation of IM and issued an information paper that recommended reclassifying first-generation IM munitions to Condition Code B: Restricted from Training Use for all DoD assets [13]. Training facilities that used the rounds were notified through a DoD directive, and demilitarization facilities were alerted to the issue. Mortar rounds containing the IHE PAX-21 were tested in March 2012, and surface residue samples were collected following high-order, single-round, blow-in-place detonations. The results of the work are summarized in Table 2. Although the organic component of the IHE functioned properly, high amounts of perchlorate and other constituent residue rates were found.
Table 2: Results of the Deposition Rates and Detonation Efficiency for the EOD Scenarios of an IM Round [14]
TOXICITY
The U.S. Army Public Health Center (USAPHC) developed a phased process to assess environmental, safety, and occupational health consequences of existing and emerging energetic material. This program assesses potential impacts to human health and environmental effects arising from exposure to energetic material, precursors, and residual contamination in soil, surface water, and ground water. Though planned demilitarization is addressed in the scope of USAPHC’s mission, emergency response performed by EOD is not.
Results for nitroguanidine (NQ), though not an IHE, are included in Table 3 for comparison. Two components found in insensitive munition formulations have limited human toxicity data, 3-nitro-1,2,4-triazol-5-one (NTO), and 2,4-dinitroanisole (DNAN), but studies involving rats and monkeys have recently been completed. The studies found that the primary adverse effect from subchronic oral NTO exposure was hypospermia, and the effects from DNAN exposure include reduced body weight, anemia, and neurotoxicity [15]. USAPHC reporting suggests that human toxicity effects from DNAN exposure are expected to be similar to most other energetic compounds and slightly less than TNT. High daily exposure to NTO affects sperm production. No chronic data are available for DNAN or NTO.
Table 3: Relative Toxicity of IMX-101 and Constituents [15]
a Median lethal dose in rats.
b BMDL10.
c Based on changes in splenic mass.
d Reproductive/developmental studies.
CONCLUSIONS
Munitions with newer, less-sensitive explosive compositions in the main charge present challenges for open-detonation disposal by using conventional EOD techniques. The lack of reliability of bare donor charge disposal techniques to consume nearly all insensitive munition fills necessitates adopting improved disposal techniques. Shaped charge attack (particularly, shaped charge jet attack) is a mature technology that represents a potential solution and is already well understood and accepted within the EOD community. Proliferating advanced shaped charge warhead design represents a threat to global security; export restrictions will likely limit availability to nonmilitary UXO disposal technicians.
Equally important, health and environmental impacts of exposure to IHE detonation products and unreacted material have not been fully explored. Continued research into potential health effects of fielded munitions and emerging insensitive energetic compounds is necessary to ensure the safety of personnel throughout the full life cycle of IM development, manufacture, use, and disposal.
References:
- NATO STANAG 2143/AEODP-10 EOD. “Principles and Minimum Standards of Proficiency,” 2014.
- SBIR STTR. “Insensitive Munitions Disposal Attack.” https://www.sbir.gov/node/372782, accessed May 2018.
- Alford Technologies. https://explosives.net/, accessed July 2018.
- Held, M. “Initierung von Sprengstoffen, ein Vielschictigen Problem der Detonationsphysik.” Explosivstoff, (translated by Lawrence Livermore National Laboratory as Ref. 02973, 1980), vol. 5, pp. 2–17, 1968.
- Patel, R. “Investigation of Possible Correlations Between Burney Constant, Held Criteria, and Critical Diameter.” ARAET-TR-06022, U.S. Army Armament Research, Development and Engineering Center, Picatinny Arsenal, NJ, January 2007.
- Voisin, M. “Critical Diameter Correlations.” MSIAC Report L-202, September 2016.
- Pope, M., and E. Baker. “Insensitive Munitions Explosive Ordnance Disposal Challenges.” The 2018 Insensitive Munitions & Energetic Materials Technology Symposium, Portland, OR, 2018.
- Tasker, D., V. Whitley, and C. Johnson. “The Interaction of Explosively Generated Plasma With Explosives.” American Institute of Physics Conference Proceedings, 2017.
- Majerus, M., and R. Brown. “Incorporation of Constant Velocity Elements in a Shaped Charge Jet for Special Applications.” The 17th International Symposium on Detonation, vol. 2, p. 185, 1998.
- Haskins, P. J., R. I. Briggs, D. W. Leeming, N. White, and P. Cheese. “Dual Fragment Impact of PBX Charges.” APS Topical Conference on the Shock Compression of Matter, 2017.
- NATO-AOP-2. “Identification of Ammunition,” 2017.
- JOTP-070. “Identification Marking for Munitions.” Joint Services Munition Safety Test Working Group, 2013.
- Walsh, M., S. Thiboutot, and B. Gullett. “Characterization of Residues From the Detonation of Insensitive Munitions: SERDP Project ER-2219,” 2017.
- Brousseau, P., S. Thiboutot, and E. Diaz. “Explosives Ordnance Disposal (EOD) of Insensitive Munitions: Challenges and Solutions.” Defence R&D Canada – Valcartier Research Center, 2018.
- Johnson, M., W. Eck, and E. Lent. “Toxicity of Insensitive Munition (IMX) Formulations and Components.” Propellants Explosives and Pyrotechnics, vol. 42, pp. 9–16, 2017.